 
Human Genetics: Concepts and Applications (Lewis), 9th EditionChapter 5:
Beyond Mendel's LawChapter OutlineCHAPTER OVERVIEW
The phenotypes of offspring do not always resemble those of the parents. As a result, the outcome of crosses can differ from predicted Mendelian ratios. This occurs for a variety of inherited traits and disorders in humans. Incomplete dominance, epistasis, penetrance, variable expression, pleiotropy, and phenocopies can affect Mendelian ratios. Mitochondria are inherited exclusively through the egg. In the case of disorders linked to defects in mitochondrial genes, the disease phenotype shows a characteristic pattern of maternal inheritance. Another variation on Mendelian inheritance occurs when genes are linked closely on the same chromosome and therefore, alleles of these genes are usually inherited together. The maternal and paternal alleles of linked genes exhibit some mixing due to crossing over during prophase of meiosis I. The frequency of recombination between alleles of linked gene increases with the distance between loci; this property has been used for gene mapping experiments. Genome-wide association studies are yielding new genetic markersCHAPTER OUTLINE5.1 A New View of Mendelian Genetics
- The traditional focus of genetics on traits determined by single genes is giving way to the realization that genes do not usually function alone.
- Most genes interact with other genes and environmental factors.
5.2 When Gene Expression Appears to Alter Mendelian Ratios- A number of factors can appear to disrupt Mendelian ratios.
Lethal Allele Combinations1. Homozygous recessive lethal alleles eliminate a progeny class.Multiple Alleles- A gene can have more than two alleles, but a diploid individual only has one or two of them.
- Different allele combinations can produce different phenotypes and different severities of symptoms.
Different Dominance Relationships- Incomplete dominance of an allele produces a phenotype in the heterozygote that is intermediate between the homozygotes.
- Codominant alleles are both expressed in a heterozygote.
Epistasis- In epistasis, one gene prevents another from contributing to the phenotype. An example of epistasis in humans is the Bombay phenotype.
- The Bombay phenotype results in O type blood for individuals who are homozygous recessive for the recessive "h" allele.
Penetrance and Expressivity- Genotypes vary in penetrance (percent of individuals with an allele who are affected) and expressivity (severity of symptoms or expression).
- Penetrance and variable expression are not well understood biochemically and are probably due to the complex biochemical environment all genes function in.
Pleiotropy- A gene with more than one phenotypic effect is called pleiotropic.
Genetic Heterogeneity- Genetic heterogeneity occurs when different genes (or alleles) cause the same phenotype.
Phenocopies- A trait caused by the environment but resembling a genetic trait is termed a phenocopy.
- It may occur in families and seem to be inherited.
The Human Genome Sequence Adds Perspective1. The Human Genome Project has enhanced our understanding of both Mendelian genetics and the extensions and exceptions to Mendel's laws.5.3 Mitochondrial Genes- Only females transmit mitochondrial genes via the oocyte.
- Traits pass to offspring of both sexes from the mother but not the father.
- Mitochondrial DNA does not cross over, mutates faster than nuclear DNA, and is present in many copies per cell.
Mitochondrial Disorders- A variety of disorders, many causing muscle weakness and fatigue, result from mutations in mitochondrial genes.
- Ooplasmic transfer has been used to overcome mitochondrial disease in children conceived in vitro.
Heteroplasmy- In heteroplasmy, a mutation is present in some mitochondria only.
Mitochondrial DNA Reveals the Past- Mitochondrial DNA can be used for forensic pedigree and phylogenetic analysis.
5.4 LinkageDiscovery In Pea Plants
- Linkage was discovered in peas when a dihybrid cross did not show a typical 9:3:3:1 ratio, but had an excess of parental types.
- Genes on the same chromosome are linked, and have different patterns of inheritance than the unlinked genes Mendel observed.
- The farther apart two genes are on a chromosome, the greater the probability that crossing over will occur between them.
Linkage Maps- Genetic linkage maps are based on the observation that the likelihood of a crossover between two linked genes is directly proportional to the distance between them.
- Linkage maps are built by tracking transmission of two traits at a time, and then determining the percent recombination between them.
Solving a Problem: Linkage- Linkage data from pooled human pedigrees can be used to develop a human linkage map.
- Linkage disequilibrium (LD) in the human genome reveals non-randomly distributed sequences where recombination occurs infrequently.
From Linkage to Genome-Wide Associations- Genome-wide association studies are yielding new genetic markers. Markers may be protein coding alleles, variable restriction sites, variable short repeated sequences, or single nucleotide polymorphisms (SNPs).
- Computer analysis of linkage data allows the computation of a LOD score (logarithm of the odds). A LOD score of 3 or greater indicates linkage between markers.
- Haplotypes are a tightly linked series of markers that appear to be inherited as a single unit.
 | 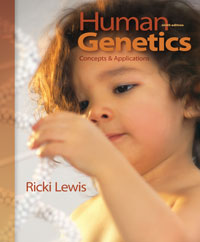 |
|